【溫馨提示】壓縮包內(nèi)含CAD圖有下方大圖片預(yù)覽,下拉即可直觀呈現(xiàn)眼前查看、盡收眼底縱觀。打包內(nèi)容里dwg后綴的文件為CAD圖,可編輯,無(wú)水印,高清圖,壓縮包內(nèi)文檔可直接點(diǎn)開(kāi)預(yù)覽,需要原稿請(qǐng)自助充值下載,所見(jiàn)才能所得,請(qǐng)見(jiàn)壓縮包內(nèi)的文件及下方預(yù)覽,請(qǐng)細(xì)心查看有疑問(wèn)可以咨詢(xún)QQ:11970985或197216396
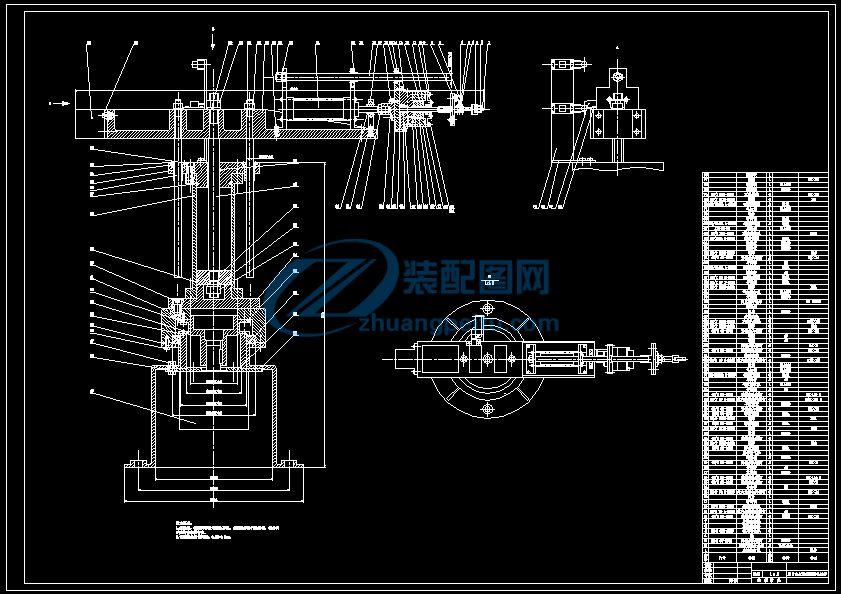



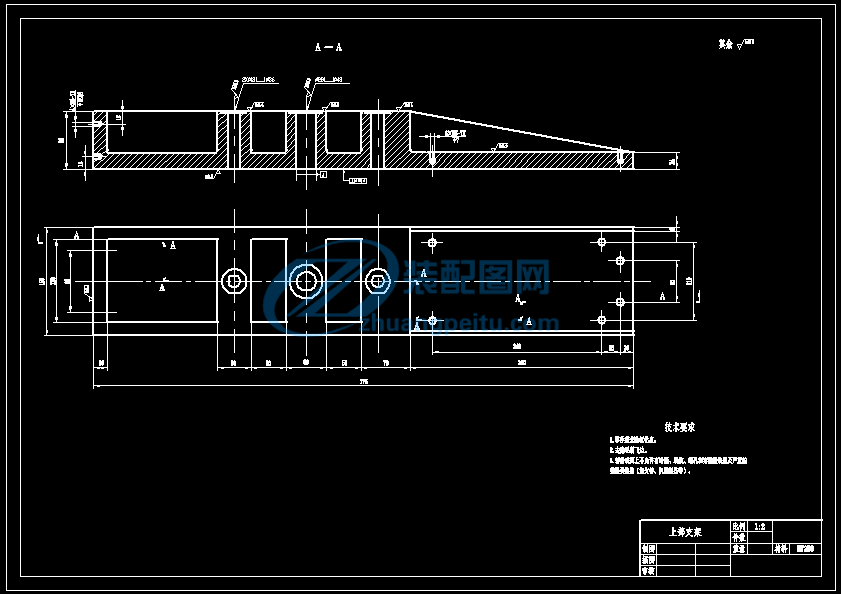
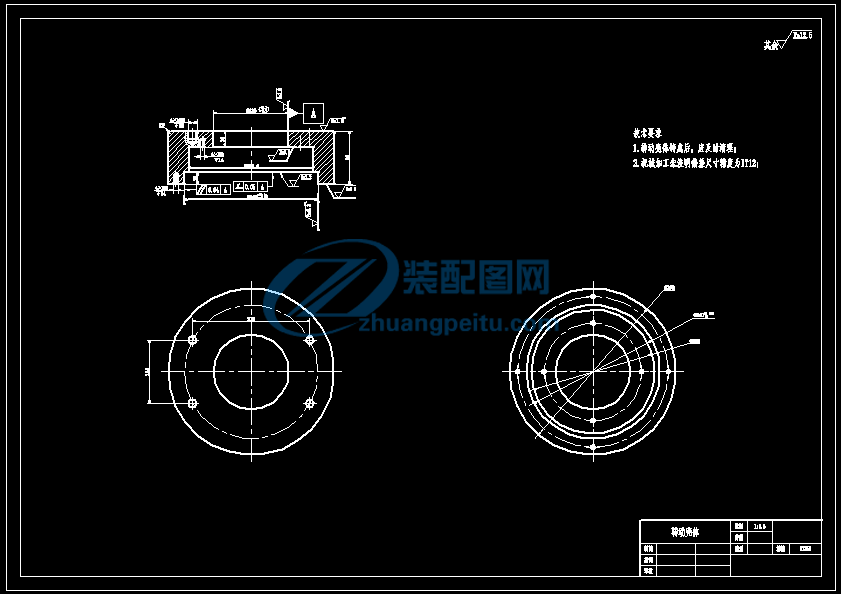

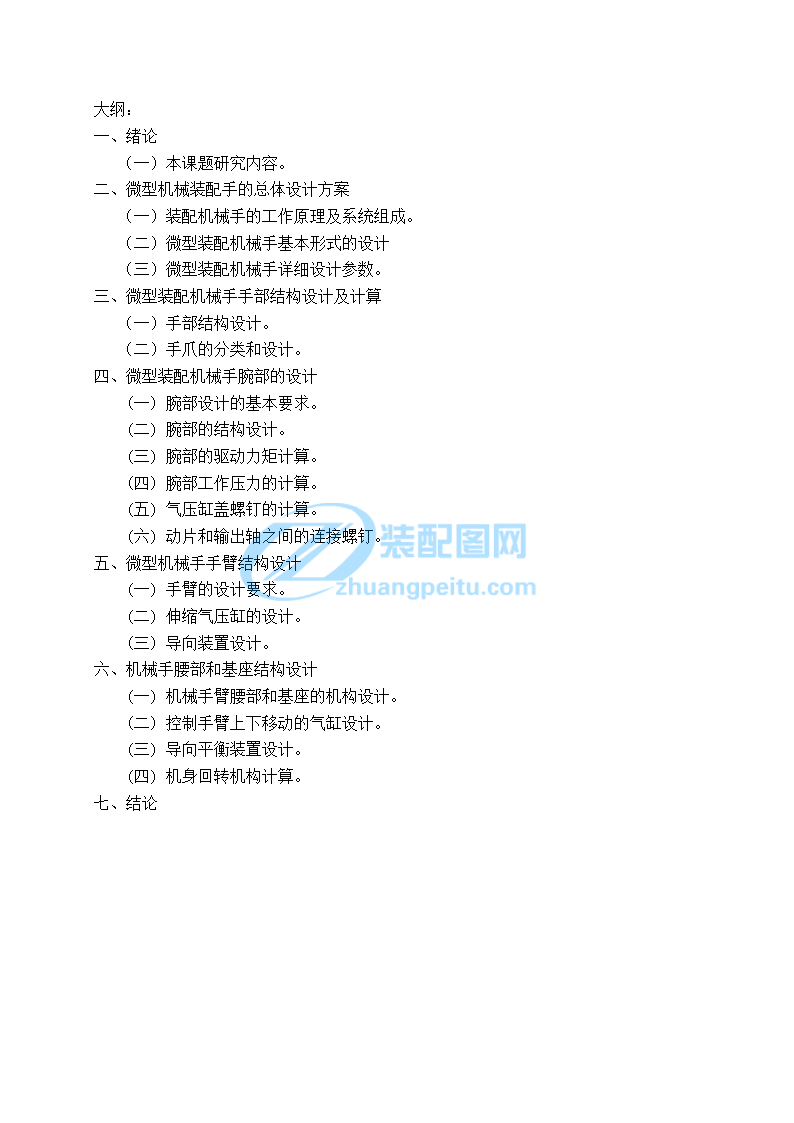

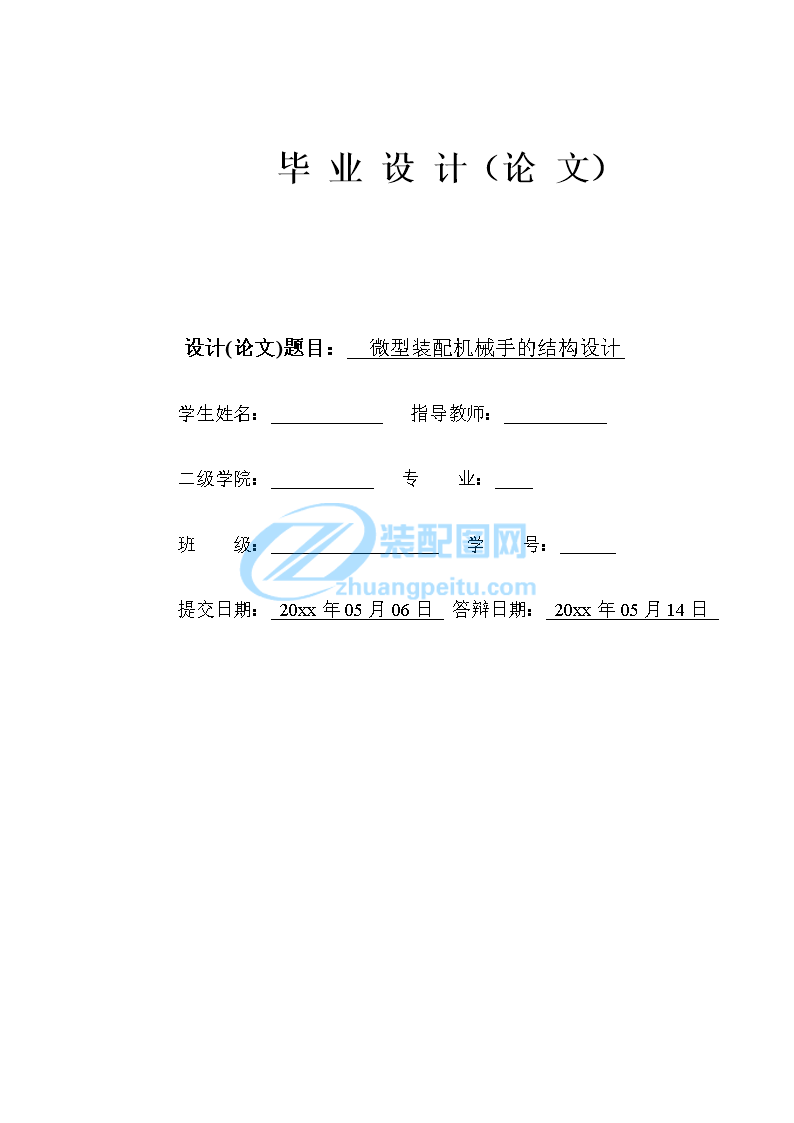
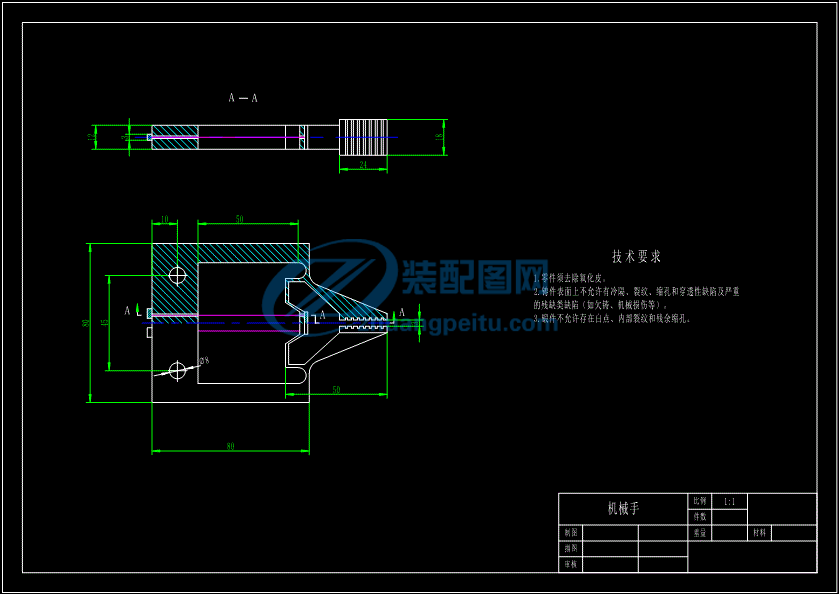
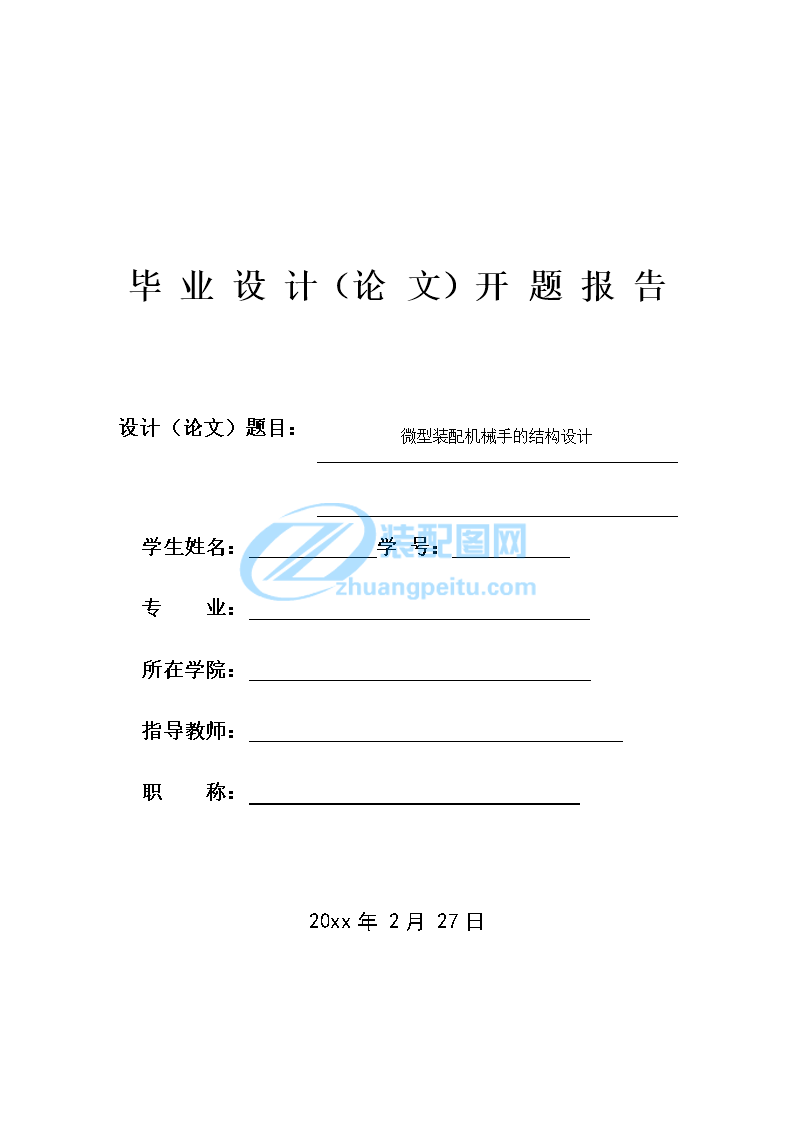



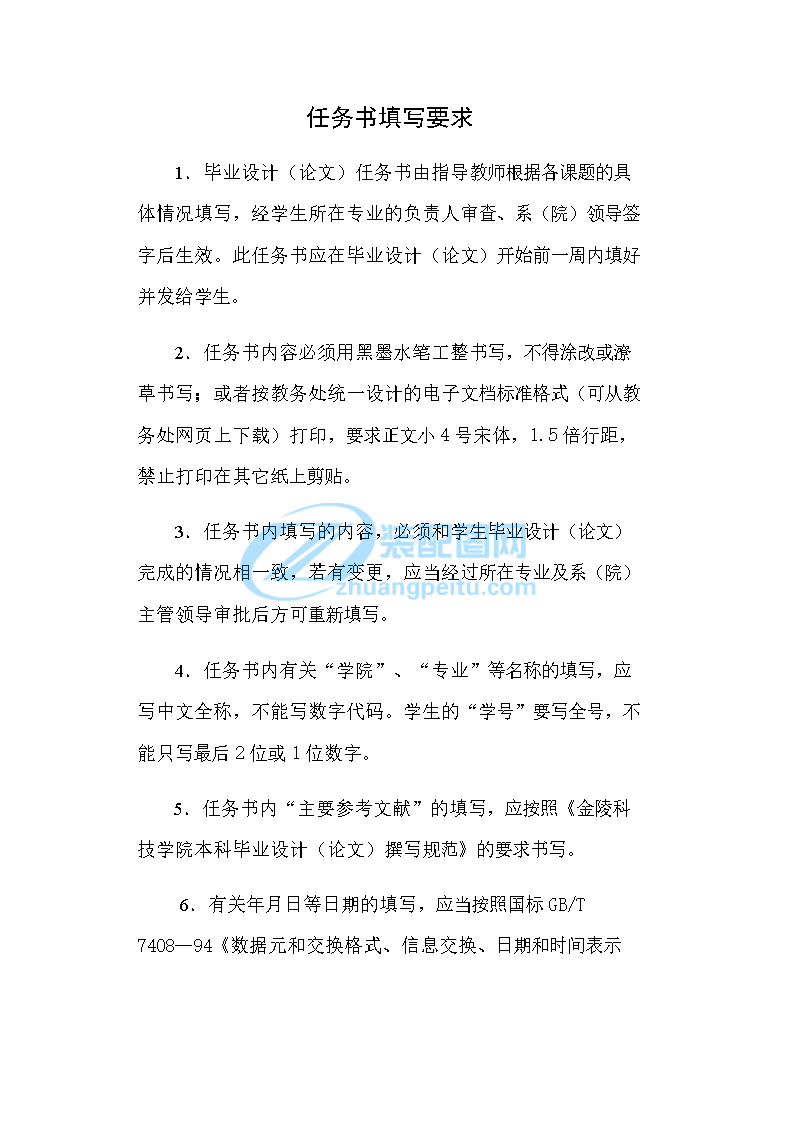

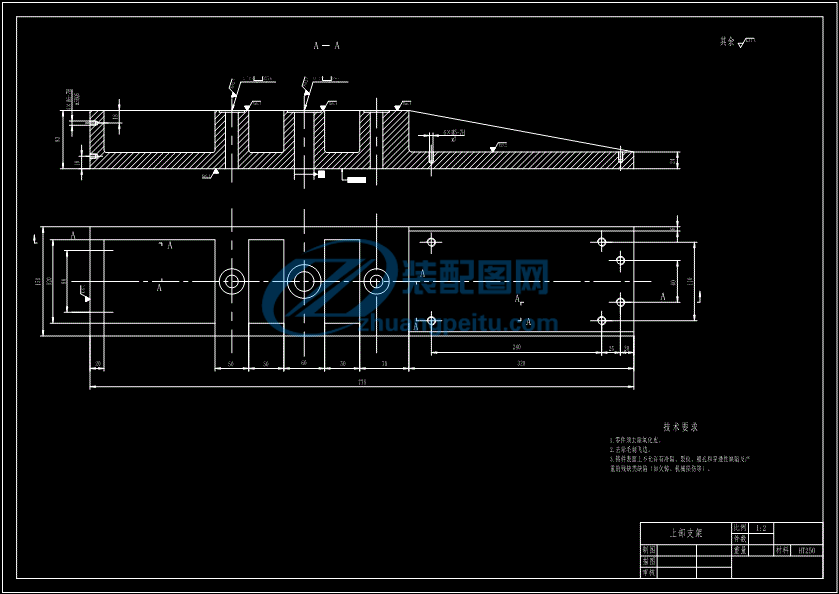
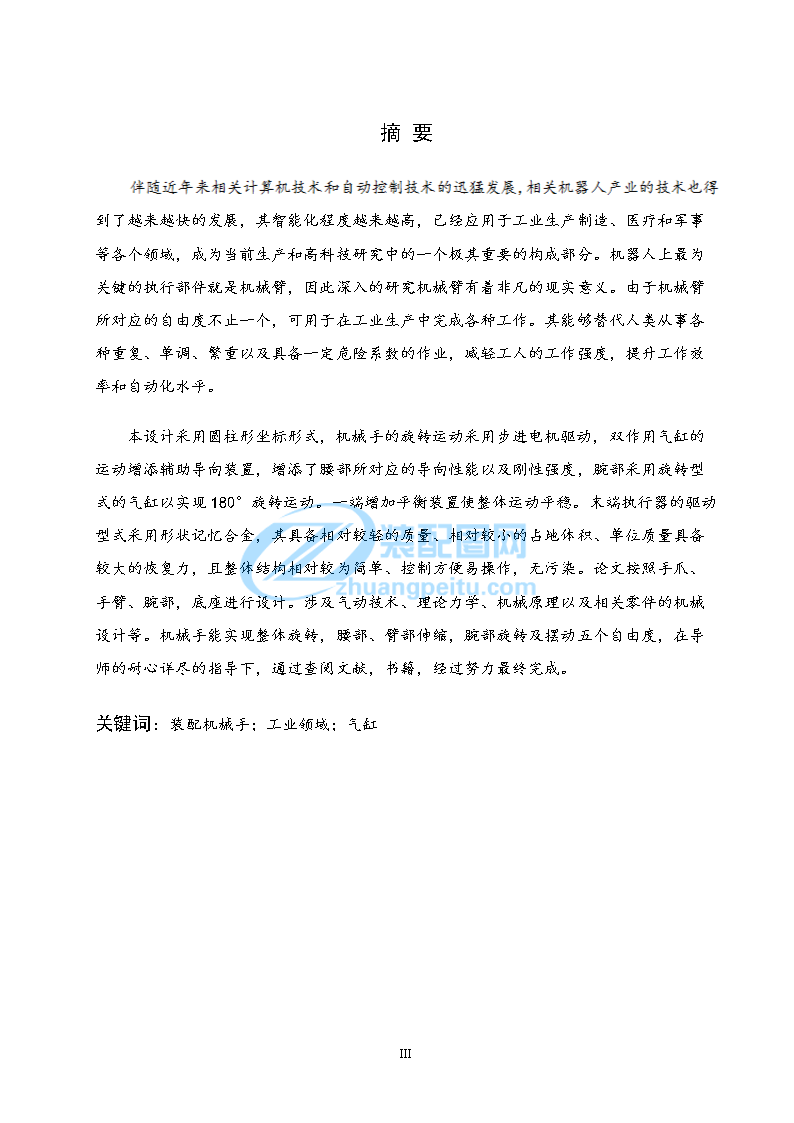






畢 業(yè) 設(shè) 計(jì)(論 文)外 文 參 考 資 料 及 譯 文
譯文題目: 微型裝配機(jī)械手的結(jié)構(gòu)設(shè)計(jì)
學(xué)生姓名: 學(xué) 號(hào):
專(zhuān) 業(yè):
所在學(xué)院:
指導(dǎo)教師:
職 稱(chēng):
20xx年 2月 27日
Impact of dynamic virtual and real robots on perceived safe waiting time and maximum reach of robot arms
Parry P.W. , Vincent G. and Gulcin
School of Industrial Engineering and Engineering Management, The Hong Kong University of Science and Technology,Clear Water Bay, Kowloon, Hong Kong SAR, China; School of Industrial Engineering, Purdue University, West Lafayette,Indiana, USA; Regenstrief Center for Healthcare Engineering, Purdue University, West Lafayette, Indiana, USA; School of Agricultural and Biological Engineering, Purdue University, West Lafayette, Indiana, USA; School of Industrial Engineering, Istanbul Technical University, Istanbul, Turkey
(Final version received February 2011)
This research examines perception of dynamic objects and robots in a virtual and real industrial work environment. The studies are modelled after those of Karwowski and Rahimi from the early 1990s. By applying virtual reality technology, the real workplace can be simulated in the virtual world for theimprovement of facility design. Perception of hazard and risk, safe waiting time, maximum reach of robot arm are measured related to the impact of parameters such as robot size, speed and type and exposure to a virtual accident. Analysis includes techniques such as sequential experiments to compare results in the virtual and real environments. These methods may be considered as a model for studying perception and transfer in other domains. The comparison of the analysed data in the virtual and real environments helps to further determinethe transferability of performance and perception from virtual reality to real. Results show similarity in perceived safe waiting time, but there are large differences in perceived maximum reach of robot arms between the virtual and real environments. Using the preliminary results from the integrated data in the sequential experiments, potential guidelines for using virtual facility layout in industry are discussed.
Keywords: human–robot interaction; perception of hazard and risk; maximum reach of robot arms; sequential experiment; data bridging
1. Introduction
Many industrial companies utilise industrial robots to perform dangerous tasks in industry to avoid possible hazards. Still, others are attempting to reduce musculoskeletal disorders through the use of hybrid automation such as assist devices (Nussbaum 2000). According to the ‘World Robotics 2007’ published by Union Nations Economic Commission from Europe (www.euron.org), there were approximately 951,000 units in 2006 and it is expected to be1,200,000 units in 2010 (Table 1).
Since the robots are used more frequently in workplaces, issues related to human–robot interaction (HRI) are more often considered by researchers and practitioners. According to Dhillon et al.(2002) survey, 523 papers about robot safety and reliability were published between 1973 and 2000. Most of this research was done between
1982 and 1990, and robot safety and reliability research has been incrementally decreased since 1986 (Dhillon et al.2002). On the other hand, between 1995 and 2000, multidisciplinary research in HRI has been more recently started by the collaboration of researchers from human factors, robotics, cognitive science, psychology and natural
language, and during that time many conferences and workshops were dedicated to HRI such as IEEE International Symposium on Robot and Human Interactive Communication (RoMan), Association for the Advancement of Artificial Intelligence’s (AAAI) Symposia Series, IEEE International Conference on Robotics and Automation(ICRA) (Goodrich 2007). Since 2006, international HRI conference has been held annually.
One of the issues related to HRI is the safety of the users. In reference to the statistics on Occupational Injuries compiled by the Labour Department of Hong Kong Government in 2007, there were 3967 injuries and 21 fatalities in the manufacturing industry in total. They indicated that there had been 491 workers injured due to striking against or being struck by dynamic moving objects (the number one type of accident) in the manufacturing industry.
1.1 Safe robot speed
The causes of accidents related to robots could be ascribed to some human perceptual, physical and psychological limitations including human perception of robot size, speed and range of motion that can affect the human behaviour (Carlsson 1984). Different speed of robots can cause different perceptions of hazards. Kulic and Croft(2006) and Ikuta et al. (2003) used velocity as an input while developing a danger index during HRI. The forceexerted by robot arms is high with fast speed of robot motion. However, it should be noted that Haddadin et al.(2007) conducted crash tests with robot and dummy head to decide the impacts of collisions between robot and human. They reported that a robot, with arbitrary mass driving moving at speeds up to 2 m/s cannot be dangerous to a non-clamped head with respect to the severity indices used in the automobile industry that are based on head acceleration. It can also be noted that other research reported that the human was not in danger for impact with the human chest, abdomen and shoulder at robot velocities up to 2.7 m/s. Beside robot velocity, robot mass’s affect on head injury criterion (HIC) was also investigated and it was reported that a heavy robot cannot pose a significant threat to the human head by means of HIC (Haddadin et al. 2008, 2009). Even though, the safe robot operating conditions (such as speed under 2.7 m/s, mechanical output under 150 N, etc.) remove physical risks, HRI stillinvolves risks related to the mental strains caused by robot motion (Aria et al. 2010).
It should be emphasised that this study is focused on cognitive aspects of robot safety. In this study, robot speeds are chosen 25 and 90 cm/s for experiments, above and below the thresholds of concern, since it was previously shown that people feel threatened by robot speed above 64 cm/s (Karwowski and Rahimi 1991). Aria et al. (2010)studied mental strains of a human operator in a cell production system where an operator assembles a product with the aid of parts feeding by the robot. Based on their physiological assessment and subjective assessment results, the operator feels discomfort when the robot’s speed is more than 500 mm/s. It can also be mentioned that the initial impact may not be the greatest reason for the concern expressed by the operators. Especially with large robots,operators are aware that the potential for a pin of body parts against other objects after impact is highly likely if collision occurs since the robot does not necessarily stop after impact whereas in an auto and in transportation,initial impact. Hence, operators’ perception of their own reaction time may be influencing their perceived safe robot speed rather than simply a concern over the damage at initial impact.
1.2 Perception of safe robot idle time
The American National Safety Standard, American National Standards Institute (1986) ANSI R15.06 was established for robot safety in the United States. Also, the Occupational Safety and Health Administration (OSHA)in the US provided guidelines for robot safety (OSHA 1987). The standards related to robotic safety are summarised in Table 2.
According to Bonney and Yong (1985) and Nagamachi (1986, 1988), the complex robot systems are potentially hazardous even in the normal mode of operation. Most accidents happened because robot operators misperceive the reasons for pauses, which are either system malfunctions or programmed stops (Sugimoto and Kawaguchi 1983).
Accident reports have shown that people can be injured or be killed by robot arms if they misperceive the work envelope and enter it during the robot operation.
1.3 Simulated accident
A simulated accident can be introduced to influence the behaviour since the expected shift in the processing ofinformation brings the task into the cognitive realm (Lehto and Papastavrou 1993, Park 1997). Rahimi and
Karwoski (1990) suggested that the idle times must be considered in designing the facility layout and robotprogrammes. It is expected that the exposure to a simulated accident will influence the waiting time to enter the
P.P.W. Ng et al.
Table 1. Robot safety standards.
work envelope for both robots. As suggested by Parsons (1986, 1987), it has been shown that the simulated robot accidents influence the robot operator after training (Karwowski et al. 1991).
Hypothesis 1
It is expected that factors of exposure to a simulated accident, size, speed and type of robots will affect waiting times(i.e. idle times) significantly in both the virtual and real environments.
1.4 Perception of maximum reach of robot arms
The robot work envelope is defined as the maximum reach of robot arms or the unsafe zone of a robot. According to Karwowski (1991), the maximum reach of robot arms were significantly affected by factors such as accident exposure, size, speeds and type of robots. The methodology from Rahimi and Karwowski (1990) and Karwowskiet al. (1991) are replicated in this study and the results will be compared.
Wright (1995) reported that real world distance perceptions are usually 87–90% of actual distances. Lamptonvirtual and real environments, but the distance in virtual were more extremely underestimated than that in real. Witmer and Kline (1998) attempted to determine how accurately stationary observers could estimate distances to objects (i.e. cylinders) in a simple virtual environment, given by static cues for distance and defined perceived distance judgement by referring to tasks in which stationary observers judge the distance between themselves and a stationary or moving object immediately perceivable to them. Based on the results from Witmer and Kline (1998), people generally underestimated distance to the objects in the virtual and real environments, but the errors in distance estimation was to be greater in virtual than that in real. Moreover, they showed that the size of the object (i.e. cylinders) influenced the estimated distance significantly but floor texture and pattern did not.
Hypothesis 2
Perception of the work envelope of the robot is related to exposure to a simulated accident, speed, types and sizes of robots in both the virtual and real industrial work environments.
2. Methods
2.1 Subjects for robot experiment
Sixty-four (32 males and 32 females) engineering students were recruited from the Hong Kong University of Science and Technology (HKUST). The subjects of the experiment had a basic understanding about robot programming and operations. The experiments took about 2 h. Each participant was paid 200 Hong Kong dollars (7.8 HKD ? 1 USD) for their participation. All participants were divided into eight groups with eight participants in each group.
2.2Equipments (robot experiment)
Two industrial robots (Yaskawa MOTOMAN-K10S and SONY SRX-410) were investigated in this research. Both robots are located in the CAD/CAM laboratory of the Hong Kong University of Science and Technology. Yaskawa MOTOMAN-K10S is a vertically articulated robot with six degrees of freedom and is mounted on the floor. Its controller is a servo-drive controlling system. The payload capacity of the robot is 10 kg. The position repeatability of the robot is 0.1 mm. The base rotation of the robot is 320 degrees about the base. The maximum reach of robot arm of MOTOMAN-K10S is 1555 mm, and the combined linear speed of all axes is 1500 mm/s. Its position repeatability is 0.1 mm.
The SONY SRX-410 is a SCARA-type high-speed assembly robot. It is a compact desktop design with four axes DC servo motor control. The work envelope of the robot is 600 mm (first arm: 350 mm; second arm: 250 mm). The maximum speed of linear motion (first and second arms combined) is 5200 mm/s. The weight of the robot is 60 kg(132.2 lbs). Its payload capacities are 5 kg (at low speed), 3 kg (at medium speed) and 2 kg (at high speed). The position repeatability of robot for the X/Y-axis and Z axis are 0.025 mm and 0.02 mm, respectively. Figures 1 and 2 show the Yaskawa MOTOMAN-K10S and SONY SRX-410 robot, respectively.
The real workplace with two robots is simulated in the dynamic virtual world by using the Virtual Reality Modeling Language (VRML). A Pentium III (600 MHz) computer with a Sony 1700 Video Display Terminal (VDT) monitor (V-frequency 75 Hz; H-frequency 60 kHz) was used and Java Script provided animation in the VRML environment. In the VRML environment, the robot motion was controlled (start and stop) through the program (buttons of control panels) using an Internet Explorer browser with Cosmo player plug-in. Since the VRML program is a virtual internet-based system, the computer is needed to connect the network to the Internet World Wide Web (WWW). Figures 3 and 4 show the MOTOMAN K10S and Sony SRX-410 robots in the virtual environment, respectively.
2.3 Experimental design
In this study, the four experiments can be divided into two main categories: (1) idle time experiment and (2) maximum reach of robot arm experiment. Each category of the experiment had two parts: one was the virtual part and the other one was the real part. All participants belonging to the real group were required to perform some virtual trials to fulfill the requirement of data bridging for the sequential experiment in order to do the common point testing. For the real group, half of the subjects (16 subjects) performed tasks in the real environment first and the other half performed some tasks in the virtual environment first. A simulated accident was shown to half of the subjects (16 subjects in the real group and 16 subjects in the virtual group).
Figur1Yaska MOTOMAN-K10S Figure 2. Sony SRX-410.
Figure 3. Virtual Yaskawa MOTOMAN-K10S Figure 4. Virtual Sony SRX-410.
2.3.1 Idle time experiment
This experiment was a six-factor ANOVA mixed design (2 genders ? 2 accident exposures ? 2 speeds ? 2 sizes ?2 types of robots ? 2 lighting levels). All independent variables had two levels. The between-subject variables are gender (males or females) and accident exposure (Yes or No). The other four within-subject independent variables are speed of the robot (10 cm/s or 90 cm/s), size of the robot (large or small), types of robots (Sony SRX-410 or Yasakawa MOTOMAN-K10S) and lighting condition (bright or dark). The dependent variable – perceived idle
International Journal of Production Research
time of robot is the time that the subjects wait as long as they feel that it is safe for them to enter the work envelope of the robot when an unexpected stop occurred that may be some trouble with the robot. The idle time of the robot is the time elapsed between the robot stop and subjects’ decision to enter the work envelope (Karwowski and Pongpatanasuegsa 1990).
2.3.2 Maximum reach of robot arm experiment
This experiment was setup considering a mixed ANOVA design. It had between-subject variables (gender and accident exposure) and within-subject variables (lighting condition, speed of robot, size of robot, type of robot and angle of approach). All factors had two levels. Perceived maximum reach of the robot arm can be defined as the maximum reach of the robot arm in relation to the actual robot envelope (Karwowski et al. 1991). The distance from the base of robot to the tip of toe of the subject was measured in the real environment by the experimenter. In the virtual environment, the internal measurement system was developed to display the X, Y and Z coordinates of viewpoints instantaneously. These coordinates can help the experimenter to calculate the accurate stopping position.
2.4 Experimental procedure
At the beginning of the experiment, subjects filled in and signed the Informed Consent Form to give their personal information (e.g. age, gender, weight and height of the subject) and guaranteed their willing and honest participation in the experiment. If any participants had problems seeing when they wore corrective lenses, then they would not be allowed to participate further in the experiment. The immersive tendency and personality of each subject were also assessed. Then, the detailed introductions about experimental procedures were explained. Industry guidelines for robot safety and safety regulations in the CAD/CAM laboratory and workshop were told to the participant. During the preview portion of the testing scenario, the participants observed robot motion in both the virtual and real environments. Also, they saw the simulated accident if the subjects belonged to the accident exposure group. These subjects could see a scenario with a virtual simulated accident that was created beforehand. In this scenario, a virtual human was knocked down by the industrial robot while it was rotating. Figure 5 shows the accident scenario after the virtual mannequin human was knocked down by the industrial robot.
2.5 Idle time experiment
2.5.1 Experiment in virtual reality
The robot motion randomly paused 10 times at four different time intervals: 5 s (4 times), 10 s (3 times), 15 s (2 times) and 20 s (1 time). For each pause, the subjects were instructed to estimate the idle times of the pause. The subject circled one answer of time estimation from the three choices: greater than 10 s, less than 10 s or cannot tell. Then, subjects were told that the next pause could be a pre-programmed stop or a stop due to system malfunction. They were required to enter the work envelope of the robot to perform the diagnostic checking. Subjects waited as long as they felt the robot would not move again. Before the subjects had actually walked into the work envelope, they verbally informed the experimenter of their decision.
In addition to measuring the time taken between the robot’s stop and subjects’ decision to enter the work envelope, the experimenter would turn-off the main power supply of the robot to ensure that the robot would not move again. Then, the subjects were allowed to enter into the work envelope of the robot. When the viewpoint of subjects moved closely towards the robots, the subjects could observe two diagnostics buttons (which indicate two characters: B and 8) placed at the base of the robot in the virtual environment. The experimenter instructed the subject to press one of these two buttons.
After the subjects pressed the button, a message was displayed on the screen that was either ‘malfunction’ or‘pre-programmed stop’. The subjects were asked to repeat the previous steps until they finished all the trials. At the end of this virtual experiment, the subjects answered additional questions to test their sense of presence.
2.5.2 Experiment in real environment
The 10 pauses at four different time intervals were randomly generated during 5 min of robot motion. After that, the subjects were instructed to estimate the time of each pause by selecting one of the three choices: greater than 10 s/less than 10 s/cannot tell. Subjects were told that the next pause could be a stop due to a system malfunction or
P.P.W. Ng et al.
Figure 5. The simulated accident scenario shows that the virtual mannequin was knocked down by the robot.programmed stop. They were also required to perform the diagnostic checking by entering the work envelope of the robot and were instructed to wait as long as they felt the robot would not move again.
Participants communicated with the experimenter verbally to inform th