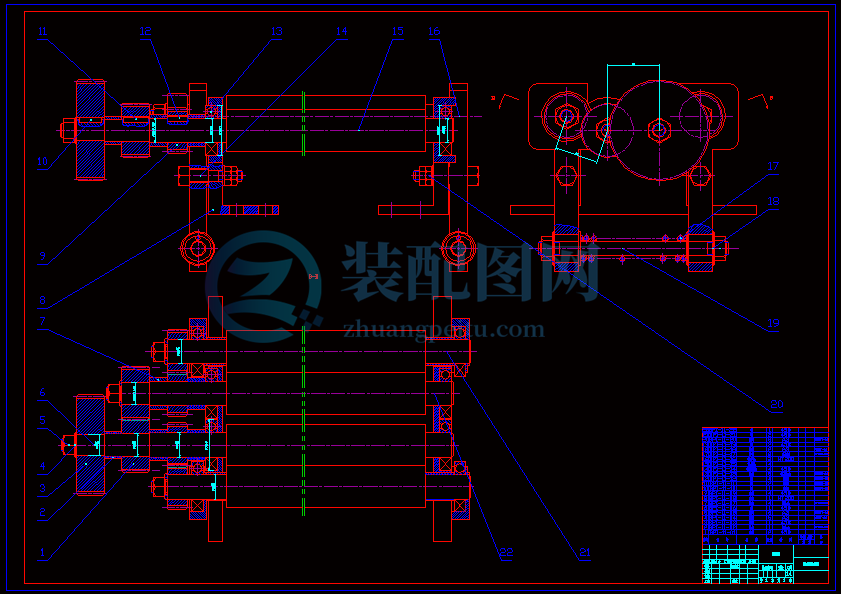




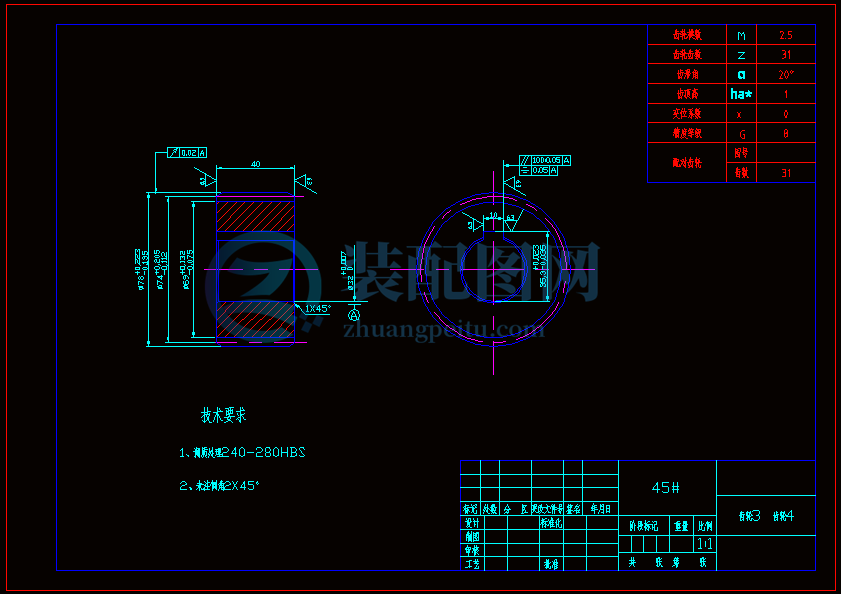
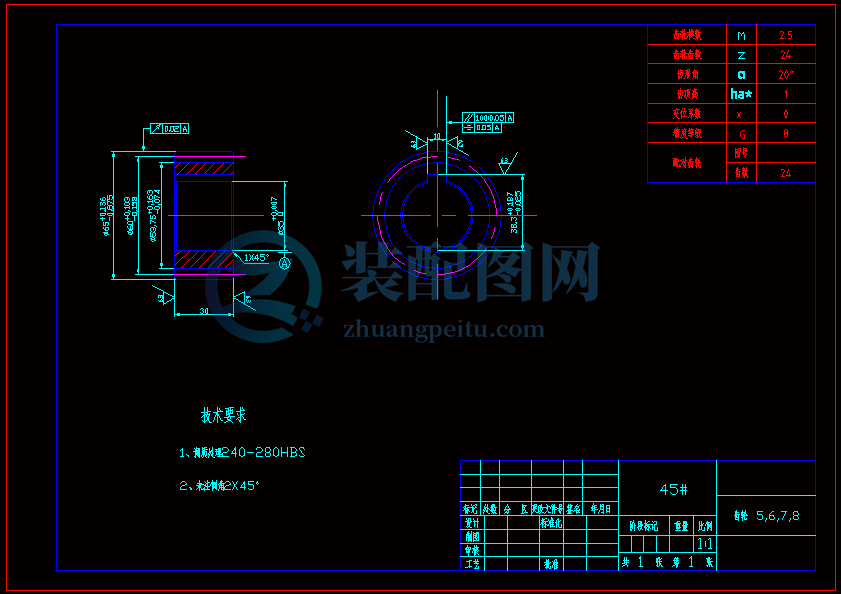
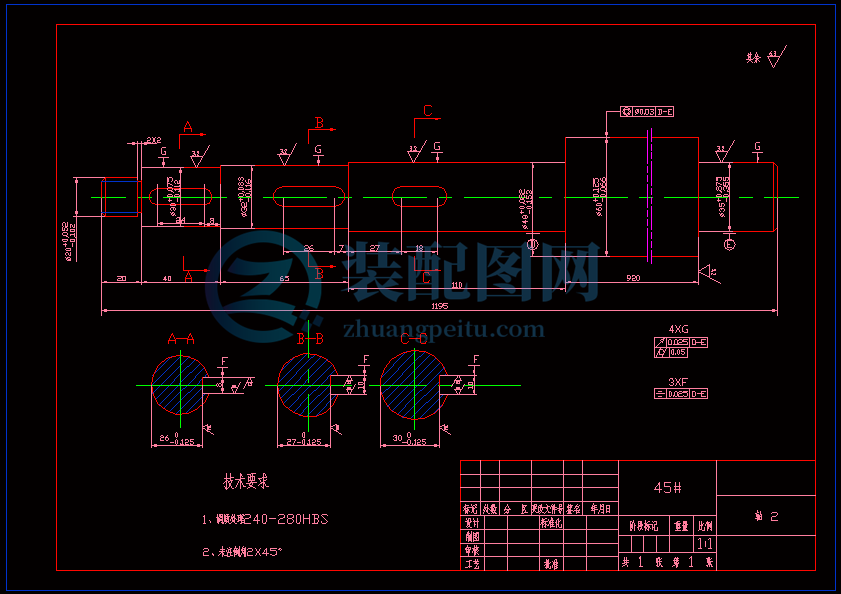
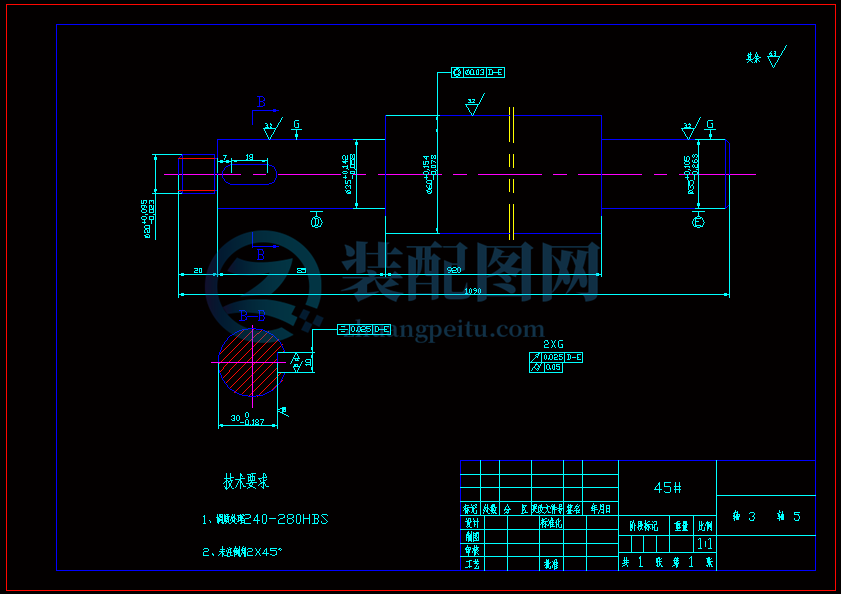
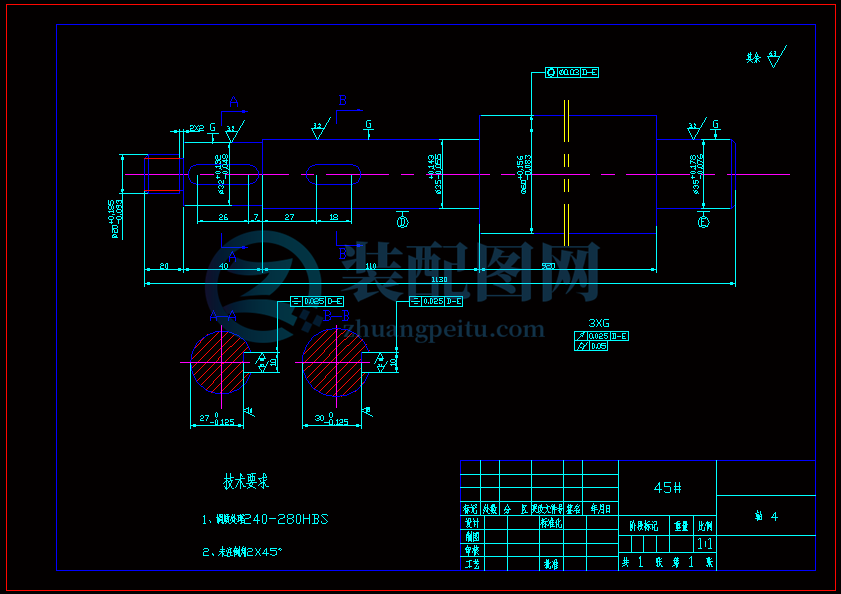

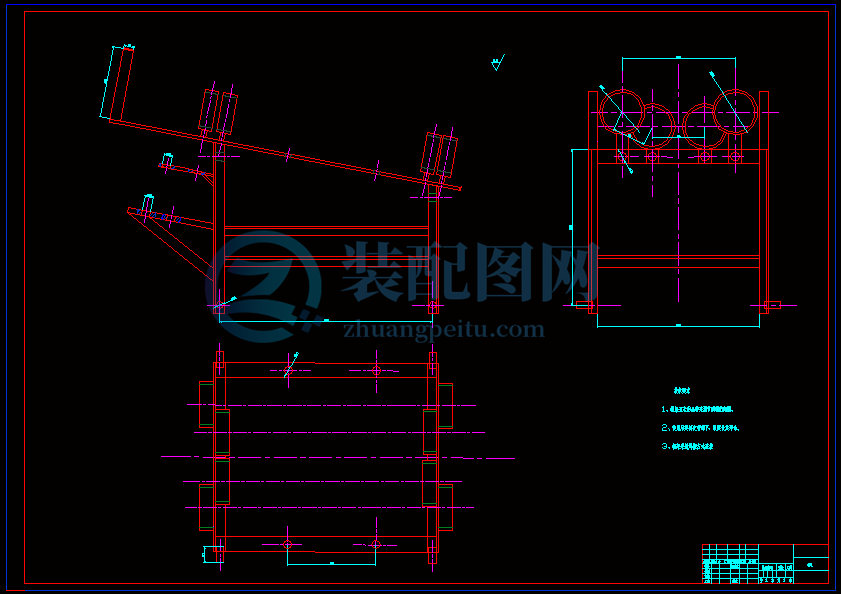
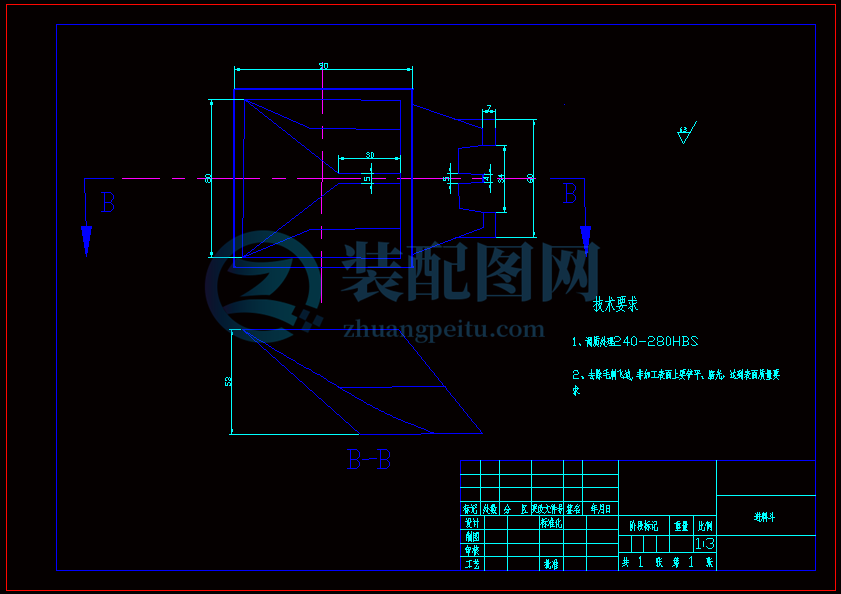
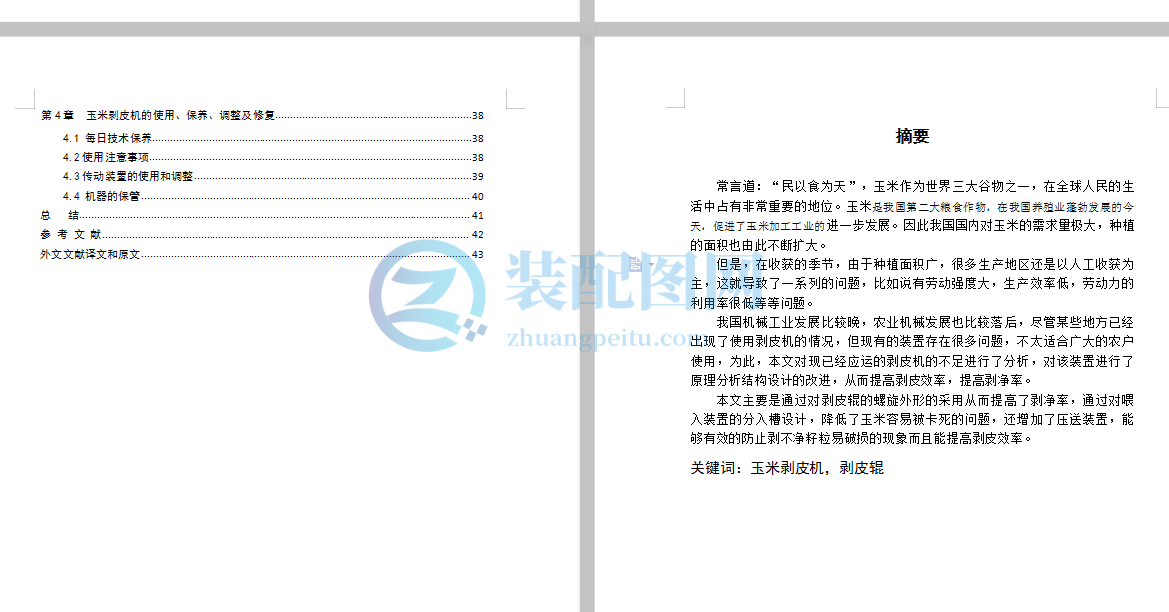
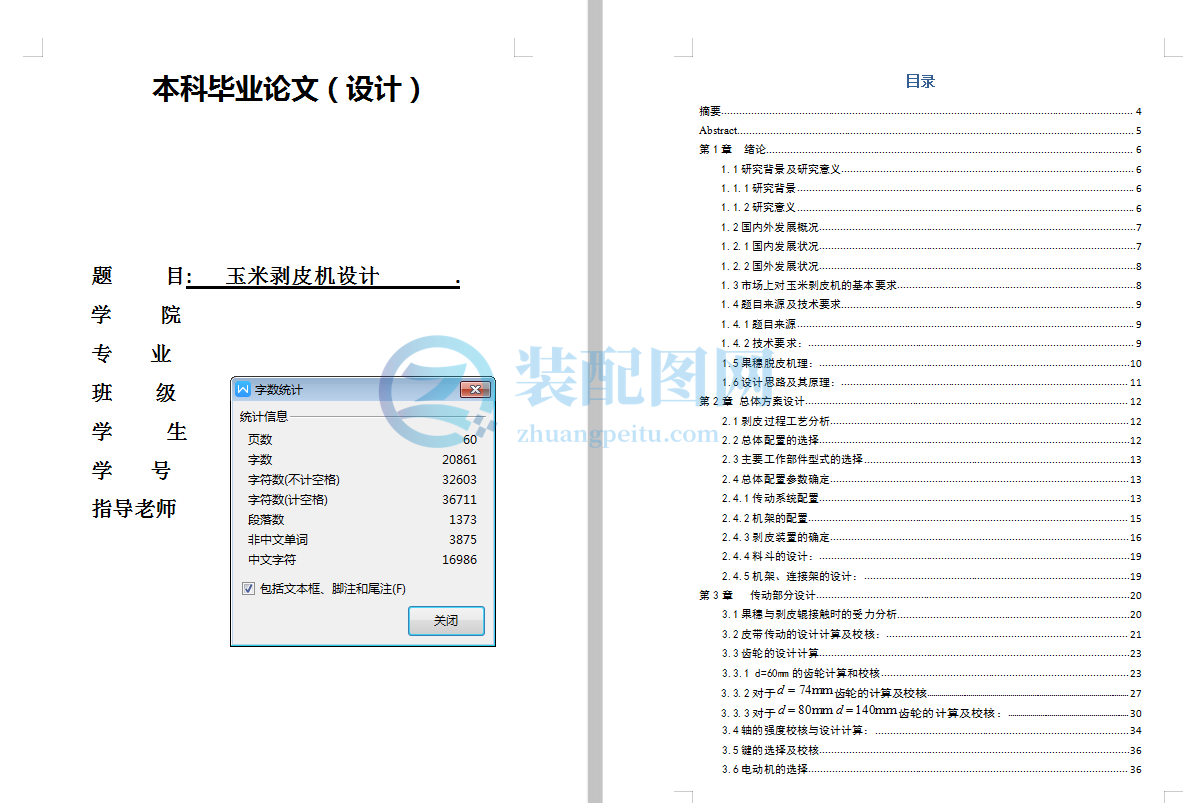
外文文獻譯文和原文
譯文:
圖 2.4.9 三個轉(zhuǎn)子觀察接近傳感器:兩個轉(zhuǎn)子橫向振動測量傳感器正交的方向和一鍵相位傳感器。示波器屏幕顯示橫向振動波形數(shù)據(jù)從兩個側(cè)面以時間為基礎的傳感器。鍵相位點的是波形上疊加。每個轉(zhuǎn)子旋轉(zhuǎn)的的鍵相位傳感器提供了序列空白/亮點。識別的方向繞在示波器屏幕上: 由于信號 X 的振幅峰值的發(fā)生較早比峰值的振幅 Y(在時間上),所述轉(zhuǎn)子的軌道是在從 X 到 Y 的方向上,獨立于轉(zhuǎn)子的旋轉(zhuǎn)方向。還提供了顯示的信號的絕對相位,以及垂直與水平運動的相對相位,相對垂直頻率與水平,并與旋轉(zhuǎn)速度。
- 19 -
圖 2.4.10 示波器的屏幕顯示與轉(zhuǎn)子的軌道運動。未經(jīng)濾波的軌道是一個 放大的轉(zhuǎn)子的中心線的橫向運動路徑。它的形狀是一個反映會發(fā)生什么的轉(zhuǎn)子。需要注意的是,如果將示波器設置在直流(dc),則軌道中心表示所述轉(zhuǎn)子的中 心線位置(例如軸承間隙內(nèi),在示波器上可以打上屏)。如果示波器上設置交流
(交流電),那么軌道中心將總是會出現(xiàn)在屏幕中間。從序列中的'空白'亮'點,創(chuàng)建疊加鍵相位信號,轉(zhuǎn)子的旋轉(zhuǎn)方向與軌道方向可以被確定。請注意,沒有關(guān) 于這個序列建立公約; 在每一個特定的情況下,它有相應的時基波(線索轉(zhuǎn)子鍵相位缺口與投影有關(guān)示波器約定)單獨進行調(diào)查。多頻振動分量,已過濾的時基信號的絕對相位,提供接近換能器的轉(zhuǎn)子觀察,“滯后”(常規(guī)正號),一個 空白(或亮)的鍵相器的點從一開始作為相位測量第一正峰值的信號。過濾組 件的轉(zhuǎn)子的橫向振動的相位表示旋轉(zhuǎn)機械中的最重要的診斷工具。鍵相傳感器 的轉(zhuǎn)子橫向振動數(shù)據(jù)聯(lián)系到其旋轉(zhuǎn)運動:
監(jiān)測旋轉(zhuǎn)機械振動
圖 2.4.11 使用鍵相位標記,以確定轉(zhuǎn)子軌道軌道旋轉(zhuǎn)頻率比,需要注意的是兩個連續(xù)的鍵相位標記之間有一個旋轉(zhuǎn)的轉(zhuǎn)子。 旋轉(zhuǎn)振動頻率比的評價
(圖 2.4.11)。鍵相傳感器所提供的信息是極其寶貴的轉(zhuǎn)子動平衡程序,在診斷其他各種機器故障,如轉(zhuǎn)子固定部分摩擦或轉(zhuǎn)子開裂是無價的。
2.4.2 傳感器的選擇
傳感器安裝在一臺機器上,是當今先進的心臟計算機監(jiān)控系統(tǒng)。機器監(jiān)控系統(tǒng)傳感器的選擇取決于機器上的建設,估計類型的振動故障和參數(shù),評估故障,機器的內(nèi)部和外部環(huán)境,轉(zhuǎn)速范圍廣,預期機動態(tài)/振動行為。機器結(jié)構(gòu)強加限制在傳感器安裝。環(huán)境參數(shù),如溫度、工作流體壓力、腐蝕性和/或輻射表示傳感器操作條件。預期的機器的動態(tài)行為,其可能的故障類型,回答問題什么參數(shù)來衡量,什么是振動信號電平,信號噪聲比和頻率范圍。
它必須被很好地理解,旋轉(zhuǎn)機械的轉(zhuǎn)子的任何表示源振動。通過測量轉(zhuǎn)子的振動,得到的直接信息。當測量外殼振動速度傳感器或加速度傳感器,振動
的信息是間接的,扭曲的外殼傳遞。這也是不完整的,不能得到轉(zhuǎn)子的軌道和內(nèi)間隙的中心線位置,在低頻率范圍內(nèi)的信號的分辨率差。
一個選擇的傳感器和進一步的數(shù)據(jù)管理系統(tǒng)可以在一個廣泛的基礎,通常, 除以旋轉(zhuǎn)機械分類,比如“關(guān)鍵的“基本”和“平衡的植物”(通用機器) 。不能幸免,大型和昂貴的機器,以及那些機器將創(chuàng)建一個重大危險源或生產(chǎn)損失, 如果他們突然變得不起作用,被列為關(guān)鍵設備。因此,主要的因素是,一個危險 的生產(chǎn)失敗的一個給定的機器。關(guān)鍵設備必須要小心使用最好的上線系統(tǒng)。另 一方面,很容易更換的通用的機器可以被周期性地監(jiān)視與可接受的結(jié)果,使用 便攜式儀器。后者可能代表接近傳感器集成到機器的振動數(shù)據(jù)的簡單收藏家, 或者他們可能會周期性地安裝在機器外殼上的速度傳感器或加速度計。
2.4.3 機床操作模式的數(shù)據(jù)采集與數(shù)據(jù)處理格式
安裝在旋轉(zhuǎn)的機器的上的的在線監(jiān)測系統(tǒng)包括換能器和的數(shù)據(jù)采集和處理 硬件和軟件。這種監(jiān)測系統(tǒng)的最終產(chǎn)品應該是人性化,充分格式化,便于在機 器健康方面的解釋。存在內(nèi)容詳實的介紹了各種格式的機器的震動和處理數(shù)據(jù), 而應收集在五個不同的機器運行狀態(tài)如下:
1. 在靜止: 這被稱為為靜態(tài)數(shù)據(jù),提供了在軸承內(nèi)部的轉(zhuǎn)子靜止位置,并可能也揭示了任何外部振動源的存在下。靜止時,各種機器元素和毗鄰的建筑,如管道,結(jié)構(gòu)共振可以進行測試,采用模態(tài)分析方法。
2. 在慢速輥,即,在低轉(zhuǎn)速(通常小于 10%的第一余額共振速度)在該狀態(tài)下, 轉(zhuǎn)子的動態(tài)響應主要是由于在轉(zhuǎn)子的弓和/或電氣和機械的跳動。慢搖數(shù)據(jù)提供 為轉(zhuǎn)子的直線度檢查,和振子/轉(zhuǎn)子表面調(diào)節(jié)檢查。
3. 在啟動時:在這段短暫的狀態(tài)振動捕獲的數(shù)據(jù)是非常重要的。它有助于確定慢輥的速度范圍內(nèi),共振速度,振動模式,自激振動的存在下,并提供了對模態(tài)的有效阻尼和同步的放大系數(shù)的信息。獲得最佳的數(shù)據(jù),如果啟動的角加速度為良好的分辨率的數(shù)據(jù)與由瞬態(tài)過程的轉(zhuǎn)動速度和低的污染是足夠小的。然而,請注意,如果機器顯示出高的振動加速度緩慢更可能危及其健康,振動源
要被淘汰。
瞬態(tài)過程的數(shù)據(jù)顯示格式是整體橫向振動的振幅,極性和過濾的 1 波特圖并過濾其他頻率成分(圖 2.4.13 到 2.4.16),與轉(zhuǎn)速轉(zhuǎn)子中心線位置(圖
2.4.17), 頻譜級聯(lián)( 圖 2.4.18 和 2.4.19 ),轉(zhuǎn)子橫向振動頻譜瀑布(圖
2.4.20 見 2.4.5 段)。全方位改善了簡單的獨立光譜波形完整顯示(圖 2.4.21 和 2.4.22),.在慢速輥,即,在低轉(zhuǎn)速(通常小于 10%的第一余額共振速度) 在該狀態(tài)下,轉(zhuǎn)子的動態(tài)響應主要是由于在轉(zhuǎn)子的弓和/或電氣和機械的跳動。 慢搖數(shù)據(jù)提供為轉(zhuǎn)子的直線度檢查,和振子/轉(zhuǎn)子表面調(diào)節(jié)檢查。兩個 XY 傳感器(參見 2.4.5 節(jié))。此信息有助于確定故障的根本原因,產(chǎn)生特定的反應模式。全頻譜圖可伴有轉(zhuǎn)子軌道和/或時基波形序列完整的顯示(圖 2.4.21 和 2.4.22) 監(jiān)測旋轉(zhuǎn)機械振動
圖2.4.12 極地塊轉(zhuǎn)子無補償(a)和(b)補償同步(1)振動數(shù)據(jù)在啟動一個橫向距離提供位移傳感器。在補償情節(jié),慢滾向量已經(jīng)矢量地減去。開始的情節(jié)已被移動到零點。該地塊上的數(shù)字代表以轉(zhuǎn)的轉(zhuǎn)速。
圖2.4.13典型波德圖(1)過濾無償振動轉(zhuǎn)子的同步,4.在操作的速度,即,在機器的動態(tài)平衡:振動信息簡稱為穩(wěn)態(tài)數(shù)據(jù)是最有意義的處理時,使用時間趨勢的格式的,以評估任何惡化的動態(tài)行為。靜態(tài)數(shù)據(jù),提供了在軸承內(nèi)部的轉(zhuǎn)子靜止位置,并可能也揭示了任何外部振動源的存在下。靜止時,各種機器元素和毗鄰的建筑,安裝在旋轉(zhuǎn)的機器的上的的在線監(jiān)測系統(tǒng)包括換能器和的數(shù)據(jù)采集和處理硬件和軟件。一個選擇的傳感器和進一步的數(shù)據(jù)管理系統(tǒng)可以在一個廣泛的基礎,通常,除以旋轉(zhuǎn)機械分類,比如“關(guān)鍵的“基本”和“平衡的植物”(通用機器) 。不能幸免,大型和昂貴的機器,以及那些機器將創(chuàng)建一個重大危險源或生產(chǎn)損失,如果他們突然變得不起作用,被列為關(guān)鍵設備。這種監(jiān)測系統(tǒng)的最終產(chǎn)品應該是人性化,充分格式化,便于在機器健康方面的解釋如管道,結(jié)構(gòu)共振可以進行測試上面的運行速度的監(jiān)測數(shù)據(jù)可以顯示在時基的波形,軌道(圖2.4.23),整體最大和最小振幅(圖2.4.24),瀑布圖(圖2.4.25),在趨勢格式的趨勢,格式包括轉(zhuǎn)子中心線位置(圖2.4.26),轉(zhuǎn)子振幅和相位.
圖2.4.14 典型的波特圖轉(zhuǎn)子同步(1)過濾補償和無償?shù)恼駝?
圖2.4.15 轉(zhuǎn)子的垂直和水平同步1x響應波特圖表示支持各向異性('分裂'共振),在低轉(zhuǎn)速的結(jié)構(gòu)共振。
圖2.4.16 極地地塊的轉(zhuǎn)子1x振動,測量內(nèi)側(cè)和外側(cè)的位置,覆蓋兩種模式: 平移和樞轉(zhuǎn)的轉(zhuǎn)子。
圖2.4.17 兩個接近傳感器配置在XY測量,并繪制隨時間變化,標志著轉(zhuǎn)子轉(zhuǎn)速和機器負荷轉(zhuǎn)子中心線位置。本特利內(nèi)華達公司診斷服務的禮貌。
圖2.4.18 振動時基信號從一個傳感器獲得的頻譜分析
圖2.4.19 頻譜級聯(lián)情節(jié)轉(zhuǎn)子振動參展1x和流體鞭振動。奇數(shù)高次諧波,諧波和/差也出現(xiàn)在頻譜中。
圖 2.4.20 (一)全光譜級聯(lián)包括流體旋轉(zhuǎn)(參見第 4 章第 4.2 節(jié)),(二)全
光譜級聯(lián)輕輕摩擦轉(zhuǎn)子在滑行過程中伴隨著一些轉(zhuǎn)子軌道(見第 5.6 章的轉(zhuǎn)子
振動 5
原文:
Figure 2.4.9 Three rotor-observing proximity transducers: two rotor lateral vibration-measuring transducers in orthogonal orientation and one Keyphasor transducer. Oscilloscope screen showing lateral vibration time-base waveform data from two lateral transducers. Keyphasor dots are super-imposed on the waveforms. The Keyphasor transducer provides the sequence of blank/bright dots at each rotor rotation. Identification of the direction of orbiting on the oscilloscope screen: Since the amplitude peak of the signal X occurs earlier (in time) than the peak
of amplitude Y, the rotor orbiting is in direction from X to Y, independently of the direction of rotor rotation. The displayed signal provides also absolute phases, as well as relative phases of vertical versus horizontal motion, relative vertical frequency versus horizontal and versus rotational speed. the rotor orbiting is in direction from X to Y, independently of the direction of rotation. The signal provides also absolute phases, as well as relative phases of vertical versus horizontal motion, relative vertical frequency versus horizontal and versus rotational speed.
Figure 2.4.10 Oscilloscope screen with the rotor orbital motion display. The unfiltered orbit is a magnified path of the rotor centerline lateral motion. Its shape is a reflection of what happens to the rotor. Note that if the oscilloscope is set on direct current (dc) then the orbit center indicates the rotor centerline position (for instance within the bearing clearance, which can be marked on the oscilloscope screen). If the oscilloscope is set on ac (alternating current) then the orbit center will always occur in the middle of the screen. From the sequence of ‘blank’ ‘bright’ spots, created by superposed Keyphasor signals, the direction of rotor orbiting versus the direction of rotation can be determined. Note that there is no established convention about this sequence; in each particular case it has to be investigated individually on the corresponding time base waves (the clues are related to rotor Keyphasor notch
versus projection and to oscilloscope convention).
frequency-multiple vibration components. The absolute phase on a filtered time- base signal, provided by a rotor-observing proximity transducer, is measured as a phase ‘‘lag’’ (conventionally with positive sign) from the start of a blank (or bright) Keyphasor dot to the first positive peak of the signal. The phases of filtered components of rotor lateral vibration represent one of the most important diagnostic tools in rotating machinery. The Keyphasor transducer ties the rotor lateral vibration data to its rotational motion: it serves
Figure 2.4.11 Using rotor orbits with Keyphasor marks to determine orbiting-to- rotation frequency ratios. Note that between two consecutive Keyphasor marks there is one rotation of the rotor. for the evaluation of vibration-to-rotation frequency ratio (Figure 2.4.11). The informa-tion provided by the Keyphasor transducer is extremely valuable for rotor balancing procedures (see Section 6.1of Chapter 6),and is priceless in diagnosing various other machine malfunctions, such as rotor-to- stationary part rubs or rotor cracking.
2.4.2 Transducer Selection
The set of transducers installed on a machine is the heart of today’s sophisticated computerized monitoring systems. A selection of transducers for the machine monitoring system depends on the machine construction, estimated types of vibrational malfunctions and parameters, which assess the malfunction, machine internal and external environ-ment, rotational speed range, and the expected machine dynamic/vibrational behavior. The machine structure imposes limitations parameters, such as temperature, working fluid pressure, corrosiveness, and/or radiation indicate the transducer operational conditions. The expected machine dynamic behavior and its possible malfunction types answer the questions regarding what parameters to measure, and what are vibration signal levels, signal-to-noise ratio
and frequency range. It has to be well understood that the rotor of any rotating machine represents a source of vibration. By measuring the rotor vibrations, direct informatio is obtained. When measuring casing vibrations using velocity transducers or accelerometers, the vibrational information is indirect, distorted by casing transmissibility. It is also incomplete, as rotor orbits and centerline positions within clearances cannot be obtained, and the signal resolution in the low frequency range is poor.
A selection of transducers and further data management systems can be made on a broad basis, generally, by dividing rotating machinery into categories, such as ‘‘critical’’, ‘‘essential’’, and ‘‘balance-of-plant’’ (general purpose machines). Large and expensive machines, which cannot be spared, as well as those machines which would create a major hazard or production loss if they suddenly became inoperative, are classified as critical machines. The main factor is, therefore,a vulnerability of production to failure of a given machine. The critical machines have to be carefully instrumented with the best on-line systems. On the other hand, the easily replaceable general-purpose machines may be periodically monitored with acceptable results, using portable instruments. The latter may represent simple collectors of vibrational data from proximity transducers incorporated into the machine, or they may be velocity transducers or accelerometers periodically installed on the machine housings.
2.4.3 Machine Operating Modes for Data Acquisition and Data Processing Formats
The online monitoring systems installed on rotating machines include transducers and data acquisition and processing hardware and software. The end product of such monitoring systems should be user-friendly, and adequately formatted for easy interpretation in terms of the machine health. There exist a
variety of informative presentation formats of the machine vibration and process data, which should be collected during five different machine operational states as follows:
1. At rest: The data, which is referred to as static data, provides the rotor static position within the bearings, and may also reveal the presence of any external source of vibration. At rest, the structural resonances of various machine elements and adjoining constructions, such as pipelines, can be tested, using modal analysis methods.
2. At slow roll, i.e., at low speed (typically less than 10% of the first balance resonance speed). In this condition, the rotor dynamic response is mainly due to rotor bow and/or electric and mechanical runout. The slow roll data serves for the rotor straightness check, and for the transducer/rotor surface conditioning check-up. The slow roll data are vital in rotor crack diagnosis and in the machine balancing process (see Sections 6.1 and 6.5 of Chapter 6). At the slow roll speed, the 1 slow roll vector can be identified and then used to compensate Bode and polar plots obtained during startup or shutdown of the machine (Figure 2.4.12).
3. At start-up: Vibrational data captured during this transient state is extremely important. It helps to identify slow-roll speed range, resonance speeds, vibration modes, presence of self-excited vibrations, and provides information on modal effective damping and synchronous amplification factors. The best data is obtained if the start-up angular acceleration is small enough for good resolution of data versus rotational speed and low contamination by transient processes. Note, however, that if the machine exhibits high vibrations the slow acceleration may even more jeopardize its health; the source of vibrations have to be eliminated.
The data display formats for transient processes are overall lateral vibration
amplitudes, polar and Bode plots of filtered 1 and filtered other frequency components (Figures 2.4.13 to 2.4.16), rotor centerline position versus rotational speed (Figure 2.4.17), spectrum cascade (Figures 2.4.18 and 2.4.19), and rotor lateral vibration full spectrum cascades (Figure 2.4.20; see subsection 2.4.5). The full spectrum is an improvement over simple independent spectra from two XY transducers (see Section 2.4.5). It provides better insight into the rotor orbital path and orbiting direction of vibration frequency components. This information helps in identification of the root cause of a malfunction generating a specific response pattern. The full spectrum plots may be accompanied by a sequence of rotor orbits and/or time-base waveforms for complete display (Figures 2.4.21 and 2.4.22).
Figure 2.4.12 Polar plots of rotor uncompensated (a) and compensated (b) synchronous (1) vibration data during start-up provided by one lateral proximity displacement transducer. In the compensated plot, the slow roll vector has been vectorially subtracted. The beginning of the plot has been moved to zero point. The numbers on the plots represent rotating speed measured in rpm.
Figure 2.4.13 Typical Bode plot rotor of synchronous (1) filtered uncompensated vibrations.
4. At operating speed, i.e., at dynamic equilibrium of the machine: The vibration information referred to as steady-state data is most meaningful when processed using time-trend formats in order to assess any deterioration in the dynamic behavior. The data monitored at the operating speed can be displayed in the time-base waveform, orbit (Figure 2.4.23), overall maximum and minimum amplitude (Figure 2.4.24), waterfall spectrum (Figure 2.4.25), and in trend formats. The trend formats include rotor centerline position (Figure 2.4.26), rotor amplitude and phase .
Figure 2.4.14 Typical Bode plot of rotor synchronous (1) filtered compensated and uncompensated vibrations.
Figure 2.4.15 Rotor vertical and horizontal synchronous 1 response plots indicating support anisotropy (‘split’ resonance) and structural resonances at low rotational speed.
Figure 2.4.16 Polar plots of rotor 1 vibrations, measured at inboard and outboard locations, covering two modes of the rotor: translational and pivotal.
Figure 2.4.17 Rotor centerline position measured by two proximity transducers in XY configuration and plotted versus time, marking rotor rotational speed and machine load. Courtesy of Bently Nevada Corporation Diagnostic Services.
Figure 2.4.18 Spectrum analysis of vibration time-base signal obtained from one transducer.
Figure 2.4.19 Spectrum cascade plot of rotor vibrations exhibiting 1 and fluid whip vibrations. Odd higher harmonics and sum/difference harmonics are also present in the spectrum.
Figure 2.4.20 (a) Full spectrum cascade of a rotor vibrations including fluid whirl (see Section 4.2 of Chapter 4). (b) Full spectrum cascade of a lightly rubbing rotor during coast down accompanied by some rotor orbits (see Section 5.6 of Chapter 5).